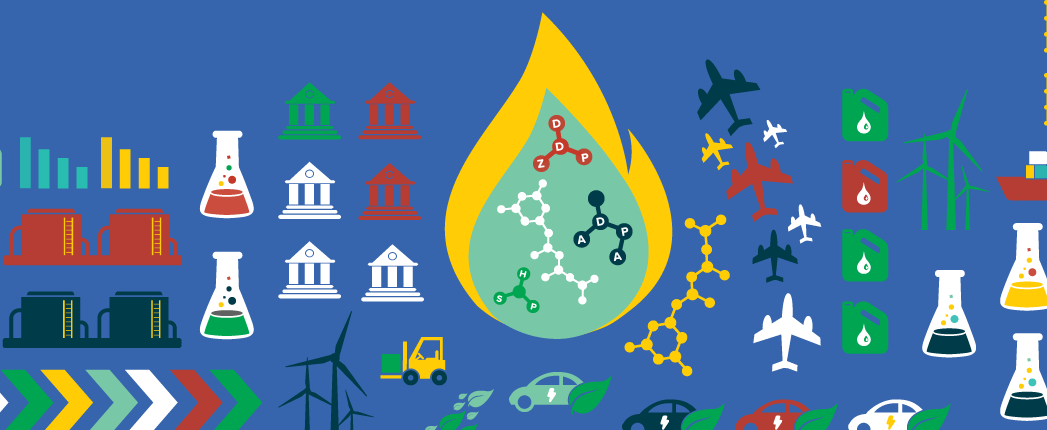
The lubricants industry isn’t static. Blenders are constantly casting an eye toward the future to formulate the next generation of lubricants. In today’s landscape, the impending dominance of electric vehicles is at the forefront of this needed innovation. But internal combustion engines can’t be written off just yet. Antioxidants have a key role to play on both fronts.
Base oils in many lubricants require antioxidants to protect them from severe oxidation and degradation. This is crucial in products like gas turbine oils, biogas engine oils and polyurea-based greases, or any other thermally stressful application.
Primary and Secondary Antioxidants
Antioxidants can be categorized as either a primary antioxidant or a secondary antioxidant. Primary antioxidants react with free radicals—reactive molecules with one or more unpaired electrons that react with other hydrocarbons—which are the primary products being formed from oil oxidation.
The first of the two primary, or “traditional,” antioxidants that currently dominate the market are aminic antioxidants. This includes alkylated diphenyl amines (ADPA), which contain a secondary amine as the functioning group.
The other primary antioxidants are phenolic antioxidants. These include simple phenolics, high molecular weight phenolics and sulfurized hindered phenols, which can also be classified as a secondary antioxidant. This category contains phenol moieties.
Secondary antioxidants decompose alkyl hydroperoxides, which are the downstream products of free radicals. Secondary antioxidants usually contain sulfur or phosphorous or both. These include zinc dialkyldithiophosphates, or ZDDP, and phosphites.
Additives Enable Innovation
Speaking at the Society of Tribologists and Lubricating Engineers’ annual meeting in Orlando, Florida, this past May, Songwon Global Technical Manager of Fuel and Lube Additives Jun Dong laid out where antioxidants fit in for the next generation of automotive lubricants.
“Most people in this room agree that the oxidation requirement for the next generation of automotive lubricants will keep going up,” Dong began.
For internal combustion engines, that means several different things in terms of trends, he continued.
The engine oils will need to endure high temperatures because modern engines are built smaller but still maintain high power output. Some are also equipped with turbo chargers. These designs mean high operating temperatures in the engine.
“Most people in this room agree that the oxidation
requirement for the next generation of automotive
lubricants will keep going up.”
— Jun Dong, Songwon |
“Second, we don’t know where the [sulfated ash, phosphorous and sulfur] level will be in next generation engine oils,” Dong explained. “But likely, this range will keep going lower.”
If that does happen, “it means the treat level of ZDDP will also go lower, and we all know that ZDDP is a highly effective antioxidant,” he said. If the level of ZDDP goes lower, it will bring additional oxidation challenges in next generation lubricants.
Next generation lubricants also means next generation standards. More stringent oxidation bench test requirements are on the horizon, and engine oils must provide longer oil service life.
Formulating lubricants for electric vehicles will provide both new and familiar challenges. These products will also need high-temperature endurance because they are fill-for-life. What’s more, the operating temperature in EV motors is very high.
“Another unique requirement for EV lubricants will be the ability to maintain dielectric and anti-corrosion properties. All of these will be impacted by oil oxidation,” Dong said. Dielectric fluids would have little or controlled electric conductivity.
Dong said Songwon, a South Korean-based chemicals manufacturer, expects traditional antioxidants to be the primary choice for the next generation of lubricants. “However, with traditional antioxidants, there’s a bottleneck,” he said. “When you keep adding higher treat level to the oil, the antioxidant efficacy will start to reach a plateau. So the question we have is, is there a way to improve or break this performance barrier by adding a small amount of booster?”
“Another unique requirement for EV lubricants will be
the ability to maintain dielectric and anti-corrosion
properties. All of these will be impacted by oil oxidation.”
— Jun Dong, Songwon |
In this case, a small amount means somewhere in the volume of 0.1% or lower.
Sulfurized hindered phenol has both primary and secondary antioxidant functionalities in one molecule, while most antioxidants have just one of these functionalities. Dong and his team ran a number of tests on base oils containing SHP to study their properties.
“The main reasons for selecting this antioxidant are potential performance due to its unique chemistry; low treat level, which is ideal as a booster for the traditional antioxidant systems comprising of amine and phenolic antioxidants; and commercial availability,” Dong told Lubes’n’Greases.
“We believe most applications use it as primary antioxidant with additional benefits of the secondary antioxidant functionalities in some formulations,” he added.
The SHP performs well regarding thermal stability, achieving 356 degrees Celsius at 50% mass loss, compared to 329 °C reached by ADPA and 315 °C for hindered phenol ester (HPE). Higher thermal stability means better durability and a better fit for high temperature applications.
Dong also examined different blends of API Group II base oils with different mixes of antioxidants to determine their performance by using a pressurized differential scanning calorimetry, a benchmark of oil oxidation.
The first two blends included one formulation with 0.5 weight percent of ADPA and another with 0.4% ADPA and 0.1% HPE. The first took 12 minutes to break 185 °C when tested, the second 14 minutes. A longer time means better performance.
The third blend contained 0.4 weight percent of ADPA, this time with 0.1 weight percent of SHP. It took 19 minutes to break the oil.
“This result clearly shows the ability of SHP to boost the performance of ADPA, and also shows a better boosting effect over the traditional hindered phenol ester,” Dong said.
Another set of blends were tested. Three blends contained 0.5% ADPA, each with varying levels of ZDDP: 0.125%, 0.25% and 0.5%. For comparison, another three blends were tested; these contained 0.4% ADPA with the same varying levels of ZDDP but with 0.1% SHP added.
These samples were measured by PDSC oxidative-induction time at 200 °C, and by a 4-ball anti-wear test.
Comparing the 0.5% ADPA with 0.125% ZDDP blend and the 0.4% ADPA, 0.125% ZDDP and 0.1% SHP blend, the former clocked in at seven minutes in the PDSC oxidative-induction test and a 0.57-millimeter wear scar in the 4-ball test. The latter went higher in the PDSC test at 12 minutes, with a wear scar of 0.5mm.
The blend with 0.5% ADPA and 0.25% ZDDP achieved 14 minutes in the PDSC test and had a 0.54-mm scar. The 0.4% ADPA, 0.25% ZDDP and 0.1% SHP blend achieved 26 minutes with a 0.5-mm scar.
Finally, there was little difference in the final pair of blends. The sample with 0.5% ADPA and 0.5% ZDDP achieved 47 minutes in the PDSC test and had a 0.53-mm scar. The sample with 0.4% ADPA, 0.5% ZDDP and 0.1% SHP reached 45 minutes with a 0.54-mm scar.
“Based on these results, there is a possibility of synergistic interactions between the ZDDP and SHP to further enhance antioxidant performance and perhaps anti-wear,” Dong said. “The SHPs also appear to favor lower levels of ZDDP in this particular base oil.”
Dong noted the base oil tested was without additives. “It looks to me that the 0.5% ZDDP in this particular system is already saturated. That’s why adding even more SHPs doesn’t do much,” he continued. “However, if you have a totally different formulation with other additives, the ZDDP will have an even harder job to deal with the decomposition of other additives. In such case, you may need to use more ZDDP, and the SHP will probably favor the higher level of ZDDP. It’s totally formulation dependent, and also probably test dependent.”
To test different formulations in lubricants, Songwon made an experimental 5W-20 passenger car motor oil pre-blend with no antioxidants. It was formulated using API Group II base oils. The formulation contained 0.04% phosphorous and 0.1% sulfur.
The baseline formulation was made by adding 0.8% ADPA and 0.2% HPE to the pre-blend. A comparative test blend was made with 0.8% ADPA, 0.19% HPE and 0.01% SHP. A second comparative blend was made with 0.8% ADPA, 0.1% HPE and 0.1% SHP.
The lubricants were deliberately under-formulated for a couple of reasons, Dong explained. First, it allows for a better assessment of antioxidant performance, and second, the company thinks the lower phosphorous and sulfur levels are representative of the formulations of the future generation of PCMOs.
These blends were put through a few ASTM bench tests and an in-house Songwon benchmark. These tests targeted different engine parts and covered the early, advanced and late oxidation stages as well as thin-film and bulk-oil oxidation.
In a thin-film oxidation uptake test (ASTM D4742), the blend with only 0.01% SHP outperformed the blend with 0.1% SHP, boosting performance by 23% compared to 11%, respectively.
“This result indicates how important it is to optimize the level of SHP for the test. Adding more isn’t always the right choice,” Dong said.
Songwon’s own bulk oil oxidation test was used to simulate the oxidation environment in the oil sump of the engine. The 0.01% SHP formulation performed identical to the baseline. The 0.1% SHP, however, improved the oxidative stability of the baseline by over 40%. It also reduced the sludge tendency of the end-of-test oils by almost 40%, while the other formulation slightly increased the sludge tendency compared to the baseline.
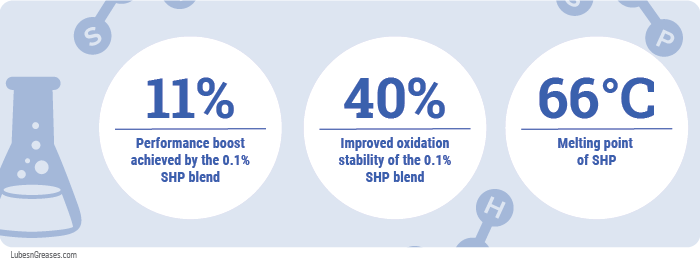
The oils were also tested using thermo-oxidation engine oil simulation tests (ASTM D7097 and D6335). Again, the lubricant with the higher SHP level outperformed the lower SHP variant in the TEOST MHT test, and significantly outperformed in the TEOST 33C test, with a 41% reduction in deposit formation as compared to the baseline.
“These results tell us that the SHP would be a very good booster for stop-and-go engine oils and hybrid applications,” Dong said.
Further testing revealed the added SHP in the blends has no adverse effects on corrosion and wear tests. Its non-corrosive nature makes SHP potentially suitable for EV lubricants like electrified transmission fluids.
To explore it further, Dong’s team took a commercial-grade ETF with only ADPA in it then made an oil that reduced the ADPA and added 0.1% SHP. The two oils showed identical electric conductivity, meaning SHP does not change the dielectrical properties of the ETF.
After accelerating the oxidation, however, the 0.1% SHP formulation showed lower electric conductivity at the same temperature than the original oil.
Dong noted that SHP has a low melting point of 66 °C, making for easier blending.
“We believe that the performance benefits shown for the SHP as an antioxidant booster will attract further attention,” Dong said.
Will Beverina is assistant editor for Lubes’n’Greases. Contact him at Will@LubesnGreases.com